How Can I Chelate Gadolinium Out of My System
Abstract
Several MRI contrast agent clinical formulations are now known to go out deposits of the heavy metal gadolinium in the brain, bones, and other organs of patients. This persistent biological accumulation of gadolinium has been recently recognized as a deleterious outcome in patients administered Gd-based contrast agents (GBCAs) for MRI, prompting the European Medicines Agency to recommend discontinuing the use of over half of the GBCAs currently approved for clinical applications. To address this problem, we find that the orally-bachelor metallic decorporation amanuensis 3,4,3-LI(i,2-HOPO) demonstrates superior efficacy at chelating and removing Gd from the body compared to diethylenetriaminepentaacetic acid, a ligand commonly used in the United States in the GBCA Gadopentetate (Magnevist). Using the radiotracer 153Gd to obtain precise biodistribution information, the results herein, supported by speciation simulations, suggest that the safe or post-hoc therapeutic use of 3,4,3-LI(1,2-HOPO) may provide a means to mitigate Gd retention in patients requiring dissimilarity-enhanced MRI.
Introduction
The utilise of gadolinium-based contrast agents (GBCAs) for magnetic resonance imaging (MRI) has been ubiquitous in radiology for virtually three decades1. Recently still, it has come to lite that the suboptimal biological stability of GBCAs can atomic number 82 to accumulation of Gd in patients' bone and brain tissue, also as to kidney damage and associated systemic conditions due to compromised renal function2,3,four. These findings have prompted a substantial volume of new reports on the identification of residual Gd accumulation in contrast MRI patients, with the emerging consensus that GBCAs of the so-called "linear" type deposit more Gd into the body than their macrocyclic counterparts (Fig. 1). In one report, the linear contrast agent Gadodiamide (Omniscan), a derivative of the diethylenetriaminepentaacetic acid (DTPA) chelator currently in utilise equally a metal decorporation amanuensisv, was found to release 24% of its Gd3+ payload afterwards only a unmarried solar day in man serum; a number that increases to 64% in the presence of elevated phosphate levels4. In July 2022 the European Medicines Agency recommended suspending the intravenous use of Gadodiamide and Gadopentetate ([Gd(DTPA)]2−, Magnevist) in light of the available data, and in September 2017, the U.South. Food and Drug Assistants's Medical Imaging Drugs Advisory Commission recommended that prescribing information should include "a warning for retentivity for all GBCAs, with greater memory of all or some of the linear GBCAs compared to the macrocyclics in certain organs including the encephalon".
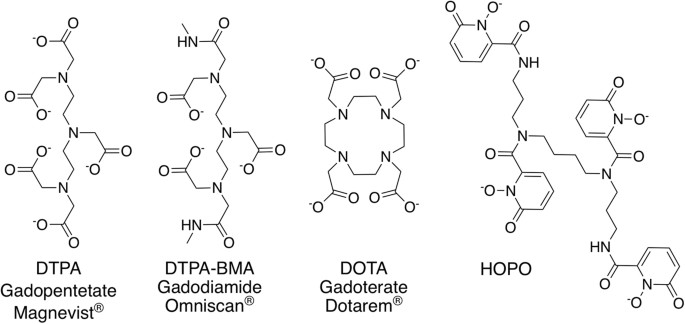
Structures of exemplary linear (DTPA, DTPA-BMA) or macrocyclic (DOTA) ligands used as GBCAs (generic and trade names are given for the corresponding Gd3+ complexes) and of the experimental chelator used in this report (HOPO).
While much of the literature has addressed both the cause and nature of metallic accumulation in vivo vi, little emphasis is placed on the prevention or remediation of Gd degradation. In this study, the chelating agent 3,iv,3-LI(ane,2-HOPO), or HOPO, is evaluated relative to DTPA equally a preventive handling for Gd accumulation (Fig. 1).
HOPO is an octadentate hydroxypyridinone ligand that is among the virtually competent chelating agents for heavy f-cake metals (log K ML at pH seven.4 for Gd of 20.five(1))7. It is orally available, non-toxic at therapeutic dosages, and is extremely selective for actinide and lanthanide ions over biologically-relevant metal ions such as iron, potassium, and calcium8. These are highly advantageous backdrop for a therapeutic chelator, and thus HOPO has been approved for a first-in-human Stage ane prophylactic trial equally an actinide decorporation agent9. This led us to surmise that HOPO could provide an effective handling for preventing and remediating the biological accumulation of Gd that has been observed in numerous recent studies. To evaluate this hypothesis, the corresponding efficacies of HOPO and DTPA as decorporation agents for the radiotracer 153Gd were probed in a murine model8,10.
Results
Bodily 153Gd Accumulation
Intraperitoneal (ip) assistants of both HOPO and DTPA was establish to promote the clearance of 153Gd, and for both chelators, the extent of clearance varies with administration fourth dimension relative to 153Gd contagion. Figure two shows the total percentage of the Gd dose recovered from the subject animals for each treatment group (%RD), equally well as the distribution of 153Gd establish in selected organ systems. In the absenteeism of treatment almost 60% of 153Gd was retained in the body after 4 days; primarily from the skeleton (41% RD), soft and abdominal tissues (4% RD), and excretory organs (liver xi% RD, kidneys 1% RD). These data reflect the established propensity of heavy metals to accumulate in the skeleton, and it is noted that this biodistribution, particularly the elevated liver aggregating of Gd compared to other non-skeletal organ systems, is consistent with previous reportseleven,12. While all treatment groups in the present study show an increase in cleared 153Gd, three are notable in their ability to reduce the amount of retained metal to under 10% RD. Treatment with DTPA 1 h prior to contamination lowered the corporeality of bodily153 Gd to nine.4 ± one.3% RD, while administration of HOPO either 24 h or i h prior to contagion reduced the corporeality of retained metal to half-dozen.iii ± one.6% RD and 1.04 ± 0.xviii% RD, respectively. The latter treatment regimen corresponds to more than than a 50-fold subtract in bodily 153Gd content compared to the control group, but more than chiefly a roughly nine-fold decrease relative to DTPA, ane of the linear GBCAs currently approved for clinical apply in the United states of america.
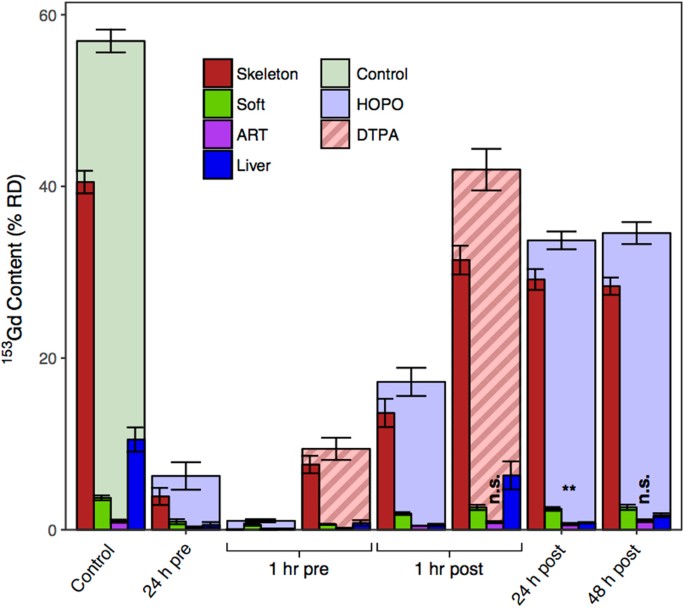
Total murine trunk content and distribution of 153Gd four days following contagion via intravenous injection into a warmed lateral tail vein, reported as % RD. Treatments were administered at a dose of 100μmol/kg via ip injection at times varying from 24 h pre- to 48 h post-Gd injection. Analyzed organ systems that comprise at least one% RD are shown. Unless otherwise indicated, the results of one-way ANOVA (Dunnett'south method) yielded p-values < 0.001 (n.south., non pregnant, **p < 0.01).
Of particular interest are the organs that exhibit substantial levels of Gd in this model, every bit well as those that show lasting Gd accumulation in man patients with potentially negative wellness outcomes: skeleton, liver, kidneys, and brain. Figures 2 and iii show that for all treatment groups except a 1 h pre-treatment with HOPO, skeletal 153Gd makes up the majority of the recovered bodily content. For that particular treatment even so, there is complete prevention of Gd accumulation in the skeleton. As previously mentioned approximately 11% RD was recovered from the liver in the control group (Tables S1, 2, Fig. 3). Interestingly, all of the treatments succeeded in reducing this burden to <2% RD except ane h mail service-contamination DTPA, where six.3 ± 1.6% RD was found in the liver (two). The substantially lower liver content of the 1 h pre-contamination DTPA treatment (0.78 ± 0.33% RD) suggests that while DTPA can effectively chelate Gd in other organ systems and prevent its accumulation in liver tissue, either it cannot effectively compete for Gd bounden with hepatic proteins or minor molecules, or it but does non reach appreciable concentrations in liver tissue. In dissimilarity, administration of HOPO even 48 h following contamination reduces the 153Gd liver content to ane.68 ± 0.24% RD, suggesting that HOPO is capable of diffusing to the highly vascularized liver tissue and as well exhibits a college affinity for Gd than exercise endogenous biomolecules.
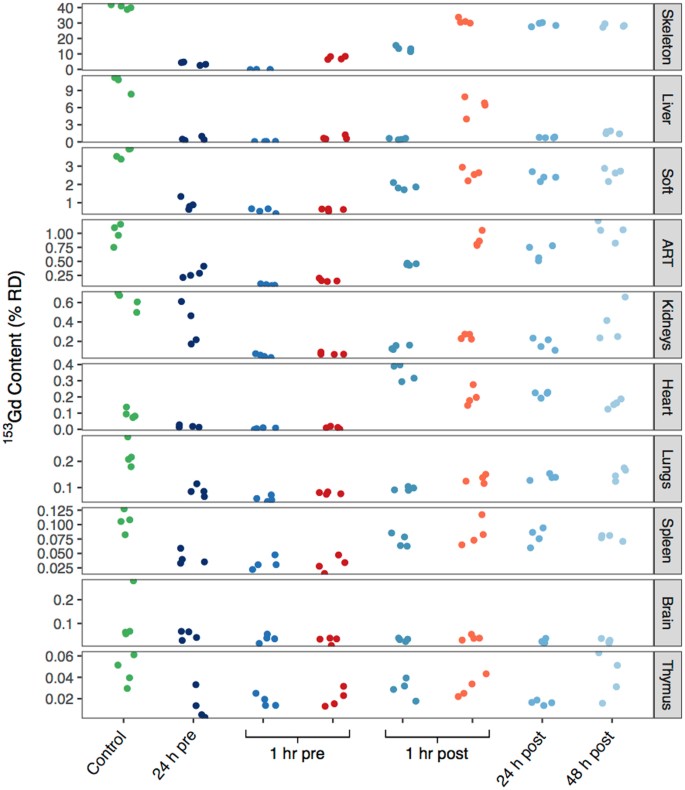
Murine organ content of 153Gd four days following contamination, reported as %RD. Data points correspond to the values obtained for each mouse in a grouping (n = 4). The information points on the correct side of the 1 hr pre and 1 hr post groups (red and orange) received treatment with DTPA, and all other non-command groups received HOPO. Organs are ranked in order of contribution to the full RD.
There is now a substantial trunk of literature implicating Gd from GBCAs in the onset of nephrogenic systemic fibrosis (NSF) in patients with suppressed renal functionthirteen,14. Thus, the aggregating of Gd in kidneys is of importance. Nephrogenic 153Gd aggregating in the control group accounts for 0.62 ± 0.09% RD, and all treatment regimens were able to promote a reduction in 153Gd content. A 1 h pre-contamination treatment of either HOPO or DTPA showed the most substantial outcome, resulting in statistically-equivalent burdens of 0.06 ± 0.02% and 0.08 ± 0.01% RD, respectively. For both chelators, delayed administration increases the 153Gd kidney concentration, suggesting that their ability to recover 153Gd is dumb over time. Given that the chelators' pharmacokinetic profiles should not be impacted by the administration time relative to contagion, the data may indicate that progressively, kidney physiology could promote formation of Gd species that are more resistant to chelation past DTPA or HOPO.
Contempo studies have also highlighted the accumulation of Gd in the brain tissue of otherwise healthy patients, and the caste to which linear and macrocyclic GBCAs atomic number 82 to such degradation remains an area of active argue3,fifteen,sixteen,17,xviii. Appropriately, the accumulation of 153Gd in the brain tissue was examined in greater particular. In the control group, an outlying data point (Fig. 3) was detected and removed (Q-value >0.95), to requite a corrected concentration of 0.063 ± 0.003% RD. This value is second merely to the thymus for the to the lowest degree amount of accumulated 153Gd, and there is no statistical difference between groups, seemingly contrary to many of the studies referenced previously. However nosotros annotation that as the timescale of intra-cranial Gd deposition is unclear, the experiment duration (4 d) was possibly also brusque to observe appreciable aggregating in the brain. Additionally, the physiological Gd concentration in the present study is at radiotracer levels; roughly 100 million times lower than therapeutic concentrations for contrast MRI. This may simply be too low to observe intracranial accumulation if there is a threshold concentration for Gd transiting the blood-encephalon barrier.
153Gd in Excreta
In addition to bodily accumulation, the excreta were nerveless daily and assayed for 153Gd content. Effigy 4 shows the 153Gd recovered from the excreta of all study groups, also equally the relative amounts found in the urine and carrion. The excreta of the control group contained 34.four% RD on 24-hour interval 1, with a roughly 95% urine and 5% feces ratio. On all subsequent days an additional ≈three% was found in the full excreta. Interestingly, while on day two an equal corporeality of 153Gd was plant in the urine and feces, on days 3 and four the ratio of excreted Gd shifted towards increased concentration in the carrion, indicating that while the urinary metabolism is responsible for the initial clearance of Gd, over time the remaining metallic is cleared through solid waste material. For the treatment groups, the cumulative full excreta on day 4 are, as expected, the inverse of the total bodily accumulations (Figure S1). The most dramatic divergence betwixt treatment groups is that upon treatment with HOPO, a substantial increase in fecal clearance is observed, whereas DTPA promotes clearance through the urinary pathway only. This is nearly clearly observed in the groups receiving HOPO 24 and 48 h mail service-contamination; those handling groups are statistically identical to the control until treatment, whereupon a significant increase in fecal excretion is establish. Information technology is noted that this is consequent with the observed chapters of HOPO to reduce liver concentrations of 153Gd when administered 48 h post-contamination. In contrast, DTPA is cleared via the renal pathway, and even a 1 h post-contagion administration leaves substantial Gd aggregating in the liver tissue.
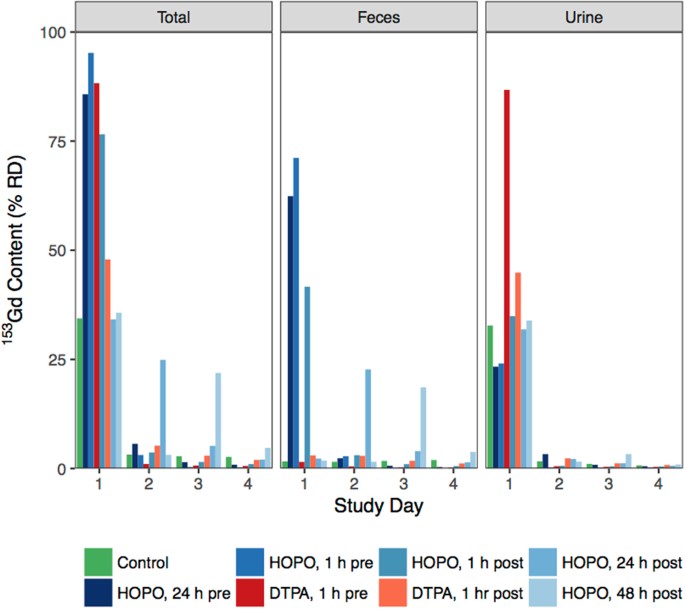
Excreted 153Gd by written report day and excretion road, reported equally %RD. Treatments were administered at a dose of 100μmol/kg via ip injection at times varying from 24 h pre- to 48 h post-Gd injection. Treatment groups administered HOPO evidence pregnant enhancement of fecal excretion relative to both control and groups receiving DTPA.
Give-and-take
Biodistribution and Decorporation
The data presented here illustrate the fundamental propensity of Gd3+ to accumulate in various organ systems, and provide insight into the strengths and weaknesses of DTPA equally a prototypical linear GBCA ligand. The chief purpose of this study was to determine the biodistribution of Gd and understand the changes engendered by two different chelating agents, and to ultimately inform the design or selection of chelators that are more ideally compatible with the biochemistry of Gd. While information technology is clearly of interest to consider the implications of these findings in light of present concerns regarding the widespread utilize of GBCAs, we caution that a few primal differences preclude directly comparing of these data to post-hoc studies of clinical subjects. First, the concentration of the 153Gd radiotracer (≈0.67 pmol/kg) was tailored to provide accurate dosimetry without unnecessary radiations exposure to either the subject animals or the researchers, and as mentioned previously the concentrations of Gd are lower than clinical doses (0.1 mmol/kg) by roughly seven orders of magnitude, adjusting for the human-equivalent dose19,20. Additionally, clinical formulations of Gd-DTPA only contain a 0.1% excess of DTPA4, even so in the present study the chelator concentrations were 100μmol/kg, a >10 one thousand thousand-fold excess. A higher level of Gd deposition can therefore exist expected in the example of an actual Gd-DTPA MRI formulation20.
Despite these deviations from clinically-relevant weather condition, the varying effects of the chelation treatments on 153Gd biodistribution give rising to of import conclusions regarding the biochemistry of Gd in various organ systems. Deposition in the skeleton is the ultimate fate of a substantial fraction of the retained contaminant. However, consistent with previous studies on the decorporation of other f-elements such as 238Pu4+21,22, 241Am3+19, or 152European union3+7, HOPO is more than constructive than DTPA at reducing the overall skeletal content of the heavy metal, even when administered upwards to 48 h mail service-contamination. In comparing, administration of DTPA only 1 h post-contamination cannot preclude Gd accumulation in the skeleton as efficiently. It is noted that our previous studies examining the chelation of 238Pu by HOPO determined that assistants even 7 d mail service-contamination was as efficacious as administration ane d post-contamination; were the present study extended further, nosotros posit that similar beliefs could be anticipated. Information technology is also probable that repeated administration of HOPO could further improve the clearance of Gd from the torso.
Synthesis of the concentration data from the liver, kidneys, and excreta derives a more comprehensive picture of the differences in pharmacokinetics between DTPA and HOPO. The inability of DTPA to retroactively clear Gd from the liver, even at 1 h post-contamination, suggests that either the biochemistry of liver tissue suppresses complexation of Gd, or that DTPA is apace metabolized via the kidneys and has negligible circulation through the liver. The almost exclusive emptying of Gd in the urine following handling with DTPA indicates that the latter is, in our stance, more than likely. In contrast, HOPO effectively removes 153Gd from the liver fifty-fifty if administered 48 h post-contamination. Coupled with the sizable increase in fecal excretion upon treatment with HOPO, as well every bit the negligible fecal clearance of the control group, the data present a compelling case that the Gd-HOPO complex is metabolized through the liver and cleared equally solid waste matter. While the utilise of linear GBCAs in patients with avant-garde kidney disease has been largely discontinued, the genesis of Gd-induced nephrotoxicity and the potential implications for otherwise healthy patients take not been established. Thus, it would seem that a decorporation strategy that does non utilize the renal excretion pathway could be advantageous.
Finally, in the present study, treatment with a chelating agent had statistically no influence on the extent of 153Gd recovered from the brain tissue; even so as discussed previously it is highly possible that either the timescale or the concentration authorities of this experiment precluded the observation of whatever treatment group-dependent trends. Intra-cranial Gd deposition has received substantial attending in the contempo literature, with mounting evidence that even in patients with uncompromised renal function, Gd will accrue in various regions of the brain1,sixteen,18,23. Additionally, efforts to narrate the speciation of Gd in both brain and other tissues accept identified insoluble Gd-containing deposits high in phosphate and calcium, strongly suggesting that Gd is no longer associated with the intended chelating amanuensishalf dozen,24. As such, it would seem imperative that more effective chelators exist employed. While the present data reveal no specific trends regarding Gd degradation in brain tissue, the overall decorporation efficacies signal that HOPO is a far better chelator of Gd compared to DTPA, particularly if the present data are indicative of delayed intracranial Gd accumulation; in this case HOPO would be expected to essentially mitigate this problem.
Gd Speciation in Physiological Conditions
In the nowadays context, the effectiveness of a chelator is determined by its ability to maintain coordination in vivo, which is of importance for preventing the precipitation of Gd deposits in the torso. To properly address this question the speciation of Gd must exist considered in the presence of not only the chelator, but as well additional biological anions capable of coordinating the metal. The in vivo chemistry of exogenous metals such as Gd is far too circuitous to be modeled completely, as it would require knowledge of the thermodynamic and kinetic backdrop of all potential ligands (small inorganic and organic molecules, transport proteins, etc.). Ideally, the effect of the ionic strength, temperature, viscosity, local concentration and interference with essential cations such equally Fe3+, Ca2+, and Zn2+ would also be taken into business relationship, but these necessary data are not known for many of the chelators utilized in GBCAs. We have therefore performed speciation simulations for the most common Gdthree+-MRI chelator systems, utilizing published thermodynamic parameters and accounting for the presence of the almost abundant and relevant natural chelators: phosphates, carbonates, hydroxides, oxalates, lactates, and citrates, too every bit the constructed chelators themselves (Table S3).
Parameterization of metal complex stability to account for chelators of different nature, acidity, and denticity in a biological surround is a nontrivial problem. To enable a direct comparing of common exogenous chelators utilized for Gd and other metals in vivo, the simulations performed here consisted of lowering the concentration of Gd and the synthetic chelator at constant pH and concentration of endogenous ligands. This aims to mimic the progressive dilution or clearance of the GBCA from the claret stream, and every bit the concentrations subtract at static weather condition of the other ligands, ultimately the chelator is unable to compete with the biological molecules and Gd is released. The concentration at which this is predicted to occur allows for the evaluation of the relative stability of the synthetic chelators post-treatment. Figure 5 shows the results of the simulations for the Gd-chelator complexes of ethylenediaminetetraacetate (EDTA), DTPA-BMA, DTPA, DOTA, and HOPO (Fig. 1), in the presence of competing biological ligands and at physiological pH. Nosotros chose DTPA-BMA, DTPA, and DOTA as models for speciation calculations in office due to the availability of thermodynamic parameters for these detail ligands, but by and large because all other currently used GBCAs are derived from these molecular structures.
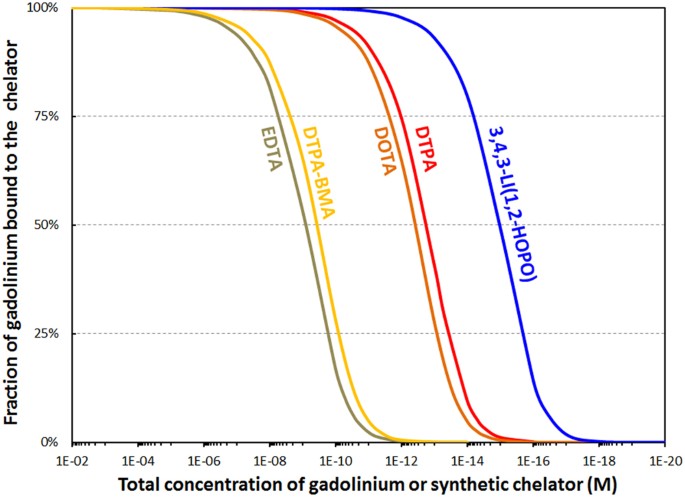
Calculated per centum of gadolinium bound to diverse chelators relevant for MRI equally a function of dilution and under biological weather condition. Full concentrations of phosphates (1.1 mM), carbonates (25 mM), oxalates (ix.2 μM), lactates (1.5 mM), and citrates (160 μM) held constant to match physiological conditions. Ratio Gd/synthetic chelator = 1.0 mol/mol. pH = 7.four. Run into Table S3 for stability constants used in these speciation simulations.
EDTA has been included because it is available and normally used for chelation therapy of atomic number 82, another heavy metal. Figure v suggests that HOPO outperforms all claiming chelators past at to the lowest degree ii orders of magnitude. In line with the in vivo studies with Gd ions, the binding power of the synthetic chelators extrapolated from the speciation simulation is as follows: EDTA = DTPA-BMA < DTPA = DOTA < HOPO. The simulation does not nonetheless account for differences in kinetic inertness betwixt chelators; while the macrocyclic DOTA chelator for instance has a lower stability abiding than DTPA, the activation free energy for demetallation is substantially greater, and DOTA has thus emerged as a more stable chelator than DTPA in vivo 4,25,26. A few boosted points from this simulation should be noted. First, while numerical values are provided on the x-axis, they should exist taken as relative stabilities rather than absolute: Other endogenous challengers (such as metal-binding proteins and other metal ions)27 are present in bodily biological media in add-on to those taken into account in the present speciation studies. Therefore the thresholds at which the synthetic chelators release Gd are expected to be higher in actuality, but this offset due to other natural ligands is expected to be very similar.
Once the Gd is released, an equilibrium is predicted between citrate, carbonate, and phosphate (Figs S3–four), nevertheless precipitation of Gd phosphate, the least soluble species, depletes that term of the equilibrium expression. In an abundance of phosphate, the equilibrium is expected to shift, inducing continued precipitation and germination of Gd phosphate deposits, every bit has been reported in the tissues of patients receiving GBCAssix,24. Further, speciation was likewise calculated for the Gd-DTPA and Gd-DTPA-BMA complexes in the presence of Ca2+ and Zn2+ ions, since the stability constants of the Ca-DTPA, Ca-DTPA-BMA, Zn-DTPA, Zn-DTPA-BMA, and the Caii+ and Zn2+ complexes of the relevant endogenous ligands are known (Table S3). The in vivo exchange of metallic ions, normally referred to in the literature as transmetallation, is a compelling theory that rationalizes the release of Gd3+ in the trunk, and our results prove an important Gd3+-Zn2+ transmetallation outcome for both linear chelators. Ca2+ is a weaker competitor for GBCAs than Zn2+ just both endogenous metals can displace Gd3+ from the pharmaceutical complexes below a certain concentration (Fig. vi, Figure S5).
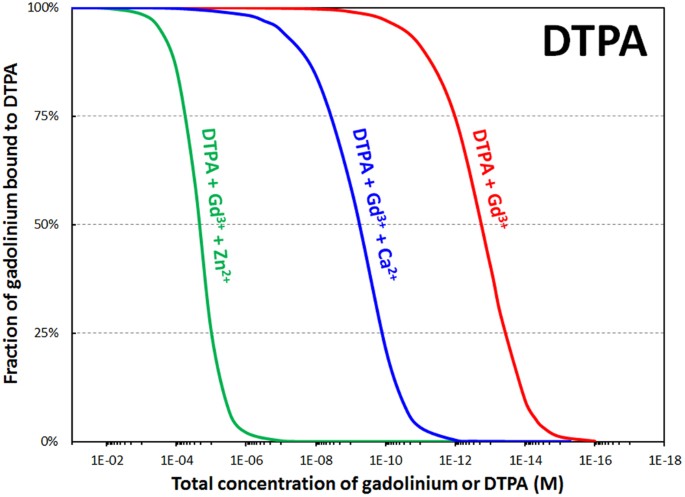
Calculated percentage of gadolinium bound to DTPA as a office of dilution in the presence of biological chelators, and with inclusion of physiological concentrations of calcium (1.1 mM) or zinc (xvμThou). Total concentrations of phosphates (1.i mM), carbonates (25 mM), oxalates (9.2 μM), lactates (one.5 mM), and citrates (160 μM) held constant to match physiological conditions. Ratio Gd/synthetic chelator = i.0 mol/mol. pH = seven.4. See Table S3 for stability constants used in these speciation simulations.
These simulations provide excellent rationale for the elevated levels of urinary Zn (zincuria) that have been observed in both human being and animal studies13, given that our findings indicate urinary clearance of the M due north+-DTPA complexes7,19,21,22. It then seems likely that Zn transmetallation of GBCAs in the kidneys could exist implicated every bit an underlying cause of NSF.
These speciation simulations were based on published thermodynamic parameters, and are to the best of our knowledge wholly supported by the available clinical data. In theory, the observed in vivo behavior of these linear GBCAs, both in terms of phosphate precipitation and Zn transmetallation, could have been predicted from such simulations prior to their clinical employ. Consideration of biologically-relevant metals every bit coordination complexes, both exogenous and otherwise, can provide rational solutions to problems such as release of Gd in vivo, utilizing existing cognition of the coordination preferences of alkali earth, transition, and heavy metals. DTPA and nigh other GBCA chelators are promiscuous in their chelation of metal ions, and the adverse chelation of Zn2+ and other vital biological cations such every bit Ca2+, Mg2+, and Fe3+ is a key reason for the inherent toxicity of DTPA and other chelators28.
In stark dissimilarity, the HOPO chelator is, past design, highly selective for lanthanide and actinide ions. This octadentate ligand binds metallic ions through the eight oxygen donor atoms of its four bidentate binding units, and is ideally suited to the larger f-block cations, while disfavoring complexation of transition metals and Group I or II ions ubiquitous in biology. In fact, the maximum tolerated dose of HOPO in rats was determined to be in excess of 100μmol kg−one d−ane over 28 days of daily oral administrationix,nineteen,22. The higher acidity of HOPO (highest p K a = 6.6) compared to DTPA (10.four) or DOTA (xi.ii) makes it more resistant to intrinsic competition between ligand protonation and metal binding at low concentrations. Due to its high denticity and steric bulk, we anticipate Gd-HOPO to be an ineffective MRI contrast amanuensis, since all coordination sites of Gd3+ should be occupied and no water molecules are expected inside the beginning coordination sphere, consistent with findings from time-resolved fluorescence measurements on the corresponding Eu3+ complex7. However, this same property makes it an excellent Gd-decorporation molecule compared to the GBCA chelators examined herein. Thus, one could envision a scenario where a currently-approved GBCA is administered, the MRI is performed, and a subsequent regimen of HOPO is prescribed to chelate and remove whatever Gd3+ that dissociates from the GBCA29,30.
In light of current information, the oral availability, safe, and selectivity for f-block elements make the metallobiochemistry of HOPO ideally suited to address the outcome of Gd accumulation in patients receiving GBCAs. Subsequent experiments volition address fundamental outstanding questions; namely, how practise the differences in chelator and Gdiii+ concentration and stoichimetry impact biodistribution, and does the co- or pre-assistants of HOPO adversely touch on the ability of GBCAs to provide the t ane relaxation enhancement needed for contrast MRI. Finally, we program to investigate if HOPO can provide improved clearance of Gd3+ from mice following dosing with clinically-relevant concentrations of linear and macrocyclic GBCAs. These studies will utilize a wider range of GBCAs and treatment conditions, every bit well every bit therapeutic levels of Gd spiked with the 153Gd radiotracer for concurrent dosimetry. Considering the many patients who accept undergone contrast MRIs and may accept bodily Gd accumulation, the present data suggest that the HOPO chelator could provide improved retroactive chelation handling compared to other ligands currently used for such applications. Previous piece of work has shown that HOPO volition circulate to the brain and bones31, and also that delayed treatment with HOPO is effective at removing 238Pu4+ from mice21. We note that the timescale of contamination relative to treatment, besides as the chemic speciation of deposited Gd, are additional factors to be explored when evaluating HOPO as a post-hoc Gd remediation therapy.
Conclusions
From the perspective of coordination chemistry, the design of a successful amanuensis for contrast MRI is somewhat paradoxical. The ligand must take sufficient affinity and selectivity for Gd so as to be stable in vivo, either from contest for Gd past endogenous ligands or transmetallation of the chelator past bioavailable metals; however, without at least one open coordination site for water to bind, t ane relaxation enhancement is quenched and the complex ceases to perform as a contrast agent. Thus, many of the all-time ligands for chelating Gd, including HOPO, may exist unsuitable equally contrast agents considering the chelator occupies all bachelor coordination sites. Recognizing the value of the clinical data afforded by contrast-enhanced MRI, such insight inspires a critical evaluation of the current strategies employed for GBCAs, and in item raises the question of whether a single-chelator arroyo is in fact the safest and most effective ways to provide MRI dissimilarity. We eagerly anticipate the creative consideration of the points raised herein, as well as the properties of f-block-specific chelators such equally HOPO, in the rapidly-expanding body of literature in this field.
Methods
Metal and Ligand Solutions
A stock solution of 153Gd-chloride in i M HCl was purchased from Eckert and Ziegler Isotope Products (Valencia, CA, Us). Contamination doses consisted of 0.two mL aliquots of solutions containing 0.925 kBq (6.94 pg) of 153Gd in 0.008 Yard sodium citrate and 0.fourteen Chiliad NaCl, pH iv. iii,iv,three-LI(1,2-HOPO) was prepared by Ash Stevens, Inc. (Detroit MI, U.s.), as described previously9. DTPA was obtained from Sigma-Aldrich (St. Louis, MO, USA) and was formulated equally Ca-DTPA using CaCO3 and NaOH, similar to the formerly available drug production commercialized past Hameln Pharmaceuticals GmbH (Hameln, Deutschland). Ligand solutions were prepared such that the dosage of 100μmol/kg was independent in 0.5 mL of 0.fourteen One thousand NaCl, with the pH adjusted to vii.4–8.four with one N NaOH. All solutions were filter-sterilized (0.22μk) prior to assistants.
Animals and Full general Procedures
All procedures and protocols used in the described in vivo studies were reviewed and approved by the Institutional Animal Care and Use Committee of Lawrence Berkeley National Laboratory, and were performed in AAALAC-accredited facilities according to prescribed guidelines and regulations. The animals were young adult (102 days old) female person (31.seven ± two.ane grand) Swiss-Webster mice (Simonsen Laboratories, Gilroy, CA, Us). Gross torso and tissue compositions, plasma, extracellular fluid, and red cell volumes of the whole body, major tissues and organs of these mice take been determined previously32. Mice were kept under a 12 h lite cycle with controlled temperature (18–22 °C) and relative humidity (30–70%), and were given h2o and nutrient advertizement libitum. Each group was housed together in a plastic stock cage lined with a 0.v cm layer of highly absorbent low-ash pelleted cellulose bedding (ALPHA-dri) for separation of urine and carrion. Intravenous (iv) injections into a warmed lateral tail vein, ip injections, and euthanasia were performed under isoflurane anesthesia. Treatment dose volumes were adjusted based on the weight of the mouse, with a 0.v mL volume respective to a 35-g mouse. To probe the effect of delayed handling, groups of iv mice were injected four with a single dose of 153Gd-citrate, and ligand or control saline solutions were administered ip in one case at the following post-contamination handling times: one h, 24 h, 48 h. To probe the consequence of safety treatment, groups of four mice were starting time administered ligand or control saline solutions ip in one case at the following pre-contagion treatment times: −one h and −24 h. Mice were and then injected iv with a single dose of 153Gd-citrate. Excreta were collected daily for iv days until scheduled necropsy (iv days afterward metallic claiming). Mice were euthanized past cervical dislocation over their respective cage to collect the excreta expelled at decease, and immediately frozen for afterwards dissection.
Tissue Sampling and Processing
Brains, thymuses, hearts, lungs, livers, spleens, and kidneys were dissected, and the abdominal balance tissue (ART, which includes intact gastrointestinal (GI) tract, reproductive organs, urinary bladder, and abdominal fat) was removed. The organ samples and partially eviscerated carcasses were managed as individual samples. Feces samples were separated manually from urine-stained cellulose bedding and treated every bit grouping samples (one group per cage). All samples were dried at 100 °C and dry-ashed at 575 °C. The ashed samples were treated with concentrated HNO3. These acidified solutions were then homogenized in dilute HNOthree and mixed with Ultima Gold (Perkin Elmer, Shelton, CT, USA) for detection by liquid scintillation counting (Packard Tri-Carb model B4430, Perkin Elmer). Additional details of autopsy procedures, tissue and excreta drove and processing, as well equally radioactivity measurements and methods of data reduction accept been previously reported for like experiments with several other long-lived radiotracers10,33.
Data management and analysis
All experiments used radioactive 153Gd as a contaminant and were managed as metabolic balance studies, in which all tissues and excreta were radioanalyzed; average radiochemical recoveries were all greater than 90% injected dose. All manipulation of the raw data was performed using the R programme. The experimental data are reported as radionuclide fractions, expressed as percent of recovered 153Gd (%RD), and both graphical and tabulated values are reported as arithmetic means ± SD. The statistical significance of changes in 153Gd concentration was determined using one-way assay of variance (ANOVA), and changes both pairwise and relative to the control group were assessed using Tukey'southward and Dunnett's tests respectively, every bit implemented in the Multcomp parcel for R. Unless otherwise noted, changes were considered significant for P < 0.001. Tests for statistical outliers were performed using Dixon's Q exam at a 95% conviction level as implemented in the Outliers bundle for R, where \({Q}=\frac{{x}_{northward}-{x}_{n-1}}{{10}_{n}-{x}_{one}}\). The R script used to perform the analysis, equally well as a complete ANOVA analysis, tin can exist establish at http://github.com/julianrees/2017_GdDecorporation.
Speciation calculations
All speciation calculations were performed using Hyperquad Simulation and Speciation software (HySS) in its high precision mode. HySS software was implemented with the published stability constants for the soluble species of Gd3+, Zn2+, and Ca2+ with the synthetic chelators of interest (DOTA, DTPA, DTPA-BMA, EDTA, and HOPO) and the endogenous chelators (hydroxides, phosphates, carbonates, citrates, lactates, and oxalates). The protonation constants of the chelators were likewise implemented in HySS software. Each speciation simulation independent betwixt forty and 74 equilibria. The stability constants used in this report are summarized in Table S3.
References
-
Kanal, E. Gadolinium based contrast agents (GBCA): Safety overview after 3 decades of clinical experience. Magnetic Resonance Imaging 34, 1341–1345 (2016).
-
Broome, D. R. et al. Gadodiamide-associated nephrogenic systemic fibrosis: Why radiologists should be concerned. American Periodical of Roentgenology 188, 586–592 (2007).
-
Kanda, T. et al. Gadolinium-based Contrast Agent Accumulates in the Encephalon Fifty-fifty in Subjects without Astringent Renal Dysfunction: Evaluation of Dissection Brain Specimens with Inductively Coupled Plasma Mass Spectroscopy. Radiology 276, 228–32 (2015).
-
Frenzel, T., Lengsfeld, P., Schirmer, H., Hütter, J. & Weinmann, H. J. Stability of gadolinium-based magnetic resonance imaging contrast agents in human being serum at 37 degrees C. Investigative radiology 43, 817–828 (2008).
-
Durbin, P. W. & Lauriston, S. Taylor Lecture: the quest for therapeutic actinide chelators. Health physics 95, 465–92 (2008).
-
Xia, D., Davis, R. Fifty., Crawford, J. A. & Abraham, J. 50. Gadolinium released from MR contrast agents is deposited in encephalon tumors: in situ demonstration using scanning electron microscopy with free energy dispersive X-ray spectroscopy. Acta radiologica (Stockholm, Sweden: 1987) 51, 1126–36 (2010).
-
Sturzbecher-Hoehne, M. et al. three,iv,3-LI(1,two-HOPO): In vitro formation of highly stable lanthanide complexes translates into efficacious in vivo europium decorporation. Dalton Transactions xl, 8340 (2011).
-
White, D. L., Durbin, P. W., Jeung, N. & Raymond, K. N. Specific sequestering agents for the actinides. sixteen. Synthesis and initial biological testing of polydentate oxohydroxypyridinecarboxylate ligands. Periodical of Medicinal Chemical science 31, 11–18 (1988).
-
Abergel, R. J. et al. Biomimetic Actinide Chelators: An Update on the Preclinical Development of the Orally Agile Hydroxypyridonate Decorporation Agents 3,4,3-LI(ane,2-HOPO) and v-LIO(Me-3,2-HOPO). Health Physics 99, 401–407 (2010).
-
Durbin, P. W., Kullgren, B., Xu, J. & Raymond, K. North. Multidentate hydroxypyridinonate ligands for Pu(IV) chelation in vivo: comparative efficacy and toxicity in mouse of ligands containing ane,2-HOPO or Me-3,2-HOPO. International journal of radiation biological science 76, 199–214 (2000).
-
Rogosnitzky, M. & Branch, Southward. Gadolinium-based dissimilarity agent toxicity: a review of known and proposed mechanisms. BioMetals 29, 365–376 (2016).
-
White, G. W., Gibby, Due west. A. & Tweedle, Grand. F. Comparison of Gd(DTPA-BMA) (Omniscan) Versus Gd(HP-DO3A) (ProHance) Relative to Gadolinium Retention in Human Bone Tissue past Inductively Coupled Plasma Mass Spectroscopy. Invest Radiol. 41, 272–278 (2006).
-
Swaminathan, S. Gadolinium toxicity: Atomic number 26 and ferroportin as central targets. Magnetic Resonance Imaging 34, 1373–1376 (2016).
-
Marckmann, P. Nephrogenic Systemic Fibrosis: Suspected Causative Role of Gadodiamide Used for Dissimilarity-Enhanced Magnetic Resonance Imaging. Journal of the American Society of Nephrology 17, 2359–2362 (2006).
-
Lancelot, E. Revisiting the Pharmacokinetic Profiles of Gadolinium-Based Contrast Agents. Investigative Radiology 51, 691–700 (2016).
-
Murata, Northward. et al. Macrocyclic and Other Non-Group 1 Gadolinium Dissimilarity Agents Deposit Low Levels of Gadolinium in Encephalon and Os Tissue. Investigative Radiology 00, 1 (2016).
-
McDonald, R. J. et al. Intracranial Gadolinium Deposition later Contrast-enhanced MR Imaging. Radiology 000, 150025 (2015).
-
Radbruch, A. et al. Gadolinium Retention in the Dentate Nucleus and Globus Pallidus Is Dependent on the Grade of Dissimilarity Agent. Radiology 275, 150337 (2015).
-
Bunin, D. I. et al. Dose-dependent efficacy and safety toxicology of hydroxypyridinonate actinide decorporation agents in rodents: towards a safe and effective human dosing regimen. Radiation research 179, 171–82 (2013).
-
Administration, U. F. & Drug. FDA Guidance. Tech. Rep., USFDA, Rockville, MD (2005).
-
An, D. D. et al. 238Pu elimination profiles after delayed treatment with iii,iv,3-LI (one,ii-HOPO) in female and male Swiss-Webster mice. International Journal of Radiation Biology xc, 1055–1061 (2014).
-
An, D. D., Kullgren, B., Jarvis, E. Eastward. & Abergel, R. J. From early prophylaxis to delayed handling: Establishing the plutonium decorporation activeness window of hydroxypyridinonate chelating agents. Chemico-Biological Interactions 267, lxxx–88 (2017).
-
Prybylski, J. P., Maxwell, Due east., Coste Sanchez, C. & Jay, M. Gadolinium degradation in the brain: Lessons learned from other metals known to cantankerous the blood–brain barrier. Magnetic Resonance Imaging 34, 1366–1372 (2016).
-
Sanyal, S., Marckmann, P., Scherer, Southward. & Abraham, J. L. Multiorgan gadolinium (Gd) deposition and fibrosis in a patient with nephrogenic systemic fibrosis - An dissection-based review. Nephrology Dialysis Transplantation 26, 3616–3626 (2011).
-
Sherry, A. D., Caravan, P. & Lenkinski, R. E. Primer on gadolinium chemical science. Periodical of Magnetic Resonance Imaging 30, 1240–1248 (2009).
-
Wang, 10. et al. A Kinetic Investigation of the Lanthanide DOTA Chelates. Stability and Rates of Formation and of Dissociation of a Macrocyclic Gadolinium(III) Polyaza Polycarboxylic MRI Contrast Amanuensis. Inorganic Chemistry 31, 1095–1099 (1992).
-
Deblonde, Yard. J.-P., Sturzbecher-Hoehne, M., Bricklayer, A. B. & Abergel, R. J. Receptor recognition of transferrin spring to lanthanides and actinides: a discriminating step in cellular acquisition of f-block metals. Metallomics 5, 619 (2013).
-
Sillanpaa, M. Environmental Fate of EDTA and DTPA. 85–111 (Springer, New York, NY, 1997).
-
Abergel, R. J., D'Aleo, A., Ng Pak Leung, C., Shuh, D. Yard. & Raymond, Thousand. N. Using the Antenna Consequence as a Spectroscopic Tool: Photophysics and Solution Thermodynamics of the Model Luminescent Hydroxypyridonate Complex [Eu 3 (3,4,3-LI(1,ii-HOPO))]. Inorganic Chemistry 48, 10868–10870 (2009).
-
Abergel, R. & Deblonde, G. Formulations and treatments employing hydroxypyridonate actinide/lanthanide decorporation agents (2017).
-
Choi, T. A. et al. Biodistribution of the Multidentate Hydroxypyridinonate Ligand [14 C]-3,4,3-LI(1,2-HOPO), a Potent Actinide Decorporation Agent. Drug Development Research 76, 107–122 (2015).
-
Durbin, P. W., Jeung, N., Kullgren, B. & Clemons, G. Thou. Gross composition and plasma and extracellular h2o volumes of tissues of a reference mouse. Health physics 63, 427–42 (1992).
-
Durbin, P., Kullgren, B., Xu, J. & Raymond, G. In Vivo Chelation of Am(III), Pu(IV), Np(V), and U(VI) in Mice by TREN-(Me-3,2-HOPO). Radiation Protection Dosimetry 53, 305–309 (1994).
Acknowledgements
This work was supported by an Innovation Grant from the Lawrence Berkeley National Laboratory, operating under U.S. Section of Free energy Contract No. DE-AC02-05CH11231.
Author information
Affiliations
Contributions
J.A.R., G.J.P.D., D.D.A. and R.J.A. designed inquiry; Thousand.J.P.D., D.D.A., C.A., S.S.G. and R.J.A. performed experimental work; J.A.R., G.J.P.D., D.D.A. and R.J.A. analyzed data; J.A.R., M.J.P.D. and R.J.A. wrote the manuscript.
Corresponding author
Ideals declarations
Competing Interests
R.J.A. and G.J.P.D are listed as inventors on two split up patent applications filed past the Lawrence Berkeley National Laboratory and describing inventions related to the inquiry results presented here.
Boosted information
Publisher's notation: Springer Nature remains neutral with regard to jurisdictional claims in published maps and institutional affiliations.
Electronic supplementary material
Rights and permissions
Open Admission This article is licensed under a Creative Eatables Attribution 4.0 International License, which permits utilize, sharing, adaptation, distribution and reproduction in any medium or format, as long every bit you give advisable credit to the original writer(s) and the source, provide a link to the Artistic Commons license, and indicate if changes were fabricated. The images or other third party material in this article are included in the commodity's Creative Commons license, unless indicated otherwise in a credit line to the material. If material is non included in the article's Creative Commons license and your intended use is not permitted by statutory regulation or exceeds the permitted use, you will need to obtain permission directly from the copyright holder. To view a copy of this license, visit http://creativecommons.org/licenses/by/4.0/.
Reprints and Permissions
Nigh this article
Cite this article
Rees, J.A., Deblonde, G.JP., An, D.D. et al. Evaluating the potential of chelation therapy to prevent and treat gadolinium deposition from MRI contrast agents. Sci Rep eight, 4419 (2018). https://doi.org/ten.1038/s41598-018-22511-vi
-
Received:
-
Accepted:
-
Published:
-
DOI : https://doi.org/10.1038/s41598-018-22511-half dozen
Further reading
Comments
By submitting a comment y'all concur to bide by our Terms and Community Guidelines. If you find something abusive or that does not comply with our terms or guidelines please flag it as inappropriate.
Source: https://www.nature.com/articles/s41598-018-22511-6
0 Response to "How Can I Chelate Gadolinium Out of My System"
Post a Comment